Note: the numbers
in square brackets refer (and link) to the List of
Publications
Biomedical Applications of Gold Nanoparticles
The uptake of metal nanoparticles by living cells is
currently a field of great research interest with significant scope for diagnostic
and therapeutic applications due to the potential of modifying the
nanoparticles with multiple functional groups, which can provide for
specific cell targeting, diagnostic functionality and/or therapeutic
effects.
Photolytic
Release of Gold Nanoparticles into the Cell Cytoplasm
Gold nanoparticles are readily taken up by cells via endocytosis, but remain located exclusively in endosomes, which limits their use for many potential
applications, since no contact with the cytoplasm or the cell nucleus is
made. We have shown that irradiation of such endosomatic
gold nanoparticles with cw-laser
light (at the wavelength of the nanoparticles’ Plasmon resonance) leads to
the partial or complete destruction of the endosome
and to the release of the nanoparticles into the cytosol
[27].
Careful choice of the irradiation conditions allows this to be achieved
without inducing cell death, providing an excellent route to deliver nanoparticles
and other agents to the cytoplasm, with the additional benefit of high
spatial and temporal control of the release.
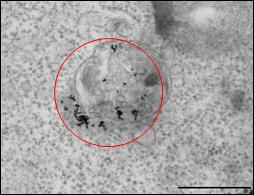
Novel
Photochemical Approach to Cancer Therapy Using Gold Nanoparticles
At higher irradiation intensities or after prolonged irradiation
of endocytosed gold nanoparticles, cell death
occurs. This opens the potential of selective cancer treatment, since
preferential uptake of nanoparticles by cancer cells can be achieved by
binding of antibody conjugated nanoparticles to malignant cells overexpressing certain biomarkers. Previously, the
mechanism of interaction of irradiated nanoparticles with cells had been
ascribed to local heating, which would affect all tissue in the irradiated
area and thus limit the specific targeting capability of nanoparticles.
However, we were able to show that irradiation of entocytosed
gold nanoparticles can lead to cell death even under
conditions where only minor heating occurs [27,
42]. This suggests the presence of a photochemical
effect which is expected to be limited to cells containing nanoparticles,
so that much higher selectivity of cell killing should be achievable. The
differentiation between photothermal or photodynamic pathways can be achieved by altering nanoparticle numbers and location and/or irradiation
conditions [42].
In fact, we have been able to show
that irradiation of gold nanoparticles with cw-laser
light leads to the formation of detectable amounts of singlet oxygen,
although the quantum yield is significantly smaller than for irradiation
with pulsed laser light [36]. In this context, we have
also carefully characterised the detection sensitivity of commonly used
singlet oxygen probes in aqueous environments [40].
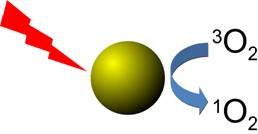
Peptide
Ligand Shells on Gold Nanoparticles
Biomimetic gold nanoparticles can be prepared using
short peptides as capping layer. These constructs combine the physical
characteristics of inorganic nanoparticles, such as their optical
properties, with the remarkable biochemical properties of proteins.
However, in general, better control over the structure of such peptide
capping layers, which is required to make full use of the potential of
these constructs, would be desirable. Using FTIR spectroscopy, we were able
to show that not only the primary sequence of the peptide, but also the
packing density and the curvature of the nanoparticle
surface affect the secondary structure of such short peptides on
nanoparticles. Thus, the CALNN peptide adopts a fully disordered structure
at lower packing density, but is forced into a straight conformation at
higher packing densities [37], independent of nanoparticle
size. In contrast, the CFGAILSS peptide can form parallel b-sheets, which are more prominent on nanoparticles
with 25 nm diameter than those with 5 nm diameter because of constraints
imposed on the peptide layer by the curvature of the nanoparticle
surface [29]. These conclusions are supported by
MD-simulations which also give more detailed insight into the structure of
the peptide capping layer [37].

a-helical peptides, on the other hand, have been shown to keep their
structure upon binding to gold nanoparticles [33].
Photodissociation of Gold Nanoparticle
Ligand Shell
We have shown that thiol-bound peptide ligands
of gold nanoparticles can be photodissociated
using short laser pulses, which in principal should allow for the
controlled release of a nanoparticle’s
"payload", for example in a cell after targeted uptake of NPs
with multiple functional ligands (antibody for targeting + drug payload).
Using femtosecond cross-correlation experiments,
we found that this effect is due to the interaction of "hot"
electrons with the thiol bond. However, the
efficiency of photodissociation is also affected
by interactions within the capping layer.
Determination
of Nanoparticle Size and Ligand Shell Thickness
Using Differential Centrifugal Sedimentation
We have developed a
sensitive method, based on DCS (Differential Centrifugal Sedimentation),
which allows the rapid determination of nanoparticle
core size as well as ligand shell thickness [33],
and have used this to study the formation of protein coronas on gold
nanoparticles [39].
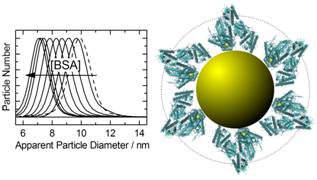
Synthesis
of Magnetic Cobalt Nanoparticles by Laser Irradiation
Magnetic nanoparticles have diverse applications in
biomedicine and as novel materials for engineering and devices, especially in
areas such as magnetic resonance imaging, targeted drug delivery,
hyperthermia treatment of solid tumours and cell separation. Their
properties depend on size and shape and on the nature of the ligands bound
to their surface. However, it is difficult to achieve a small nanoparticle size with standard synthetic methods.
We have succeeded in synthesising monodisperse
cobalt nanoparticles with less than 5 nm
diameters, using short laser pulses to stimulate the rapid decomposition
of cobalt carbonyl in a solution of stabilizers [26].
By controlling the reaction conditions, i.e. ligand concentration and
wavelength of light, it is possible to control size and size distribution
of the nanoparticles. In particular, we found that light pulses at 355
nm yielded larger nanoparticles with a broad distribution of sizes, whereas
light pulses at 266 nm resulted in smaller and monodisperse
nanoparticles, which are of particular interest for practical applications.
The different results most likely are due to the transient heating of the
solution which occurs upon irradiation at 266 nm in addition to photolysis
of cobalt carbonyl, which may cause a short burst of nanoparticle
nucleation, followed by a slower growth phase.
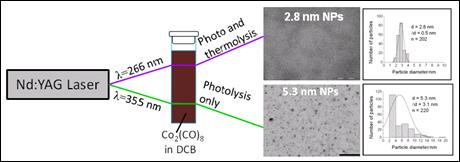
|